Thermodynamics – class 11 chemistry notes
Last updated on January 16th, 2022 at 10:56 am
This post covers Thermodynamics with class 11 chemistry notes. The branch of science which deals with the study of different forms of energy and their interconversion is called thermodynamics. Thermodynamics means the flow of heat.
- Thermodynamics is one of the fundamental subject in physical chemistry
- It’s a science of macroscopic properties i.e. properties of matter in bulk
- It deals with energy changes accompanying all types of physical and chemical processes
- It predicts the feasibility or spontaneity of a process, including a chemical reaction, under a given set of conditions
- It helps to determine the extent to which a process can proceed before attainment of equilibrium
- Thermodynamics – Some basic terms and concepts
- Types of Systems
- Macroscopic Properties of the System
- Thermodynamic equilibrium
- Types of Processes
- Common modes of transfer of energy between system and surroundings
- Internal Energy (U)
- Change of Internal Energy U of the system
- Work done (w) in various processes
- First Law of Thermodynamics
- Heat (q) in various processes
- Work, Heat & Internal energy in different processes (Infographic tabular data)
Thermodynamics – Some basic terms and concepts
System and Surroundings
System. A specified part of the universe that is under observation is called the system.
Surroundings. The remaining portion of the universe which is not a part of the system is called the surroundings. In other words, surroundings include everything other than the system. The system and the surroundings together constitute the universe.
System + Surroundings = Universe
The system and the surroundings are separated by real or imaginary boundaries. The boundary also defines the limits of the system. The system and the surroundings can interact across the boundary.
Types of Systems
Systems may be classified into three types depending upon the movement of matter and energy in or out of the system.
(i) Open system
A system that can exchange matter as well as energy with the surroundings is called an open system.
For example, hot coffee in an open flask is an example of an open system because it can gain or lose matter as well as energy.
Similarly, if we carry out a reaction between zinc granules and hydrochloric acid in an open beaker, it constitutes an open system because hydrogen evolved during the reaction (mass) goes out from the system to the surroundings and at the same time heat evolved during the reaction escapes from the system to the surroundings.
(ii) Closed system
A system that can exchange energy but not matter with its surroundings is called a closed system.
Hot coffee in a stainless flask is an example of a closed system because energy can be gained or lost (through the steel walls) but matter can’t. Similarly, all chemical reactions carried out in closed containers are examples of closed systems.
For example, the heating of hydrogen iodide in a sealed glass bulb constitutes a closed system because there is an exchange of heat energy from surroundings to the system but no exchange of matter takes place.
(iii) Isolated system
A system that can neither exchange matter nor energy with the surroundings is called an isolated system. There is no perfectly isolated system.
However, a system that is completely sealed to prevent inflow or outflow of matter and is thermally insulated to prevent the flow of heat can be considered as an isolated system.
For example, hot coffee in a corked thermos flask is an example of an isolated system.
Similarly, all chemical reactions carried out in closed containers insulated from the surroundings are examples of isolated systems.
All physical and chemical processes taking place in open in our daily life are open systems because these are continuously exchanging matter and energy with the surroundings.
Macroscopic Properties of the System
Thermodynamics deals with matter in terms of bulk (a large number of chemical species i.e. atoms, molecules, or ions) behavior.
The properties of the system which arise from the bulk behavior of matter are called macroscopic properties. The common examples of macroscopic properties are pressure, volume, temperature, surface tension, viscosity, density, refractive index, etc.
State of a System
The state of a system means the condition of existence of the system when its macroscopic properties have definite values. If any of the macroscopic properties of the system change, the state of the system is also said to change. Thus, the state of the system is fixed by its macroscopic properties.
State Variables
The measurable properties required to describe the state of the system are called state variables. For example, temperature, pressure, volume, composition, etc. are the state variables.
State Functions
A state function is a property of the system whose value depends only upon the state of the system and is independent of the path or manner by which the state is reached.
The change in the value of these properties (state functions) depends only upon the initial and final states of the system and not on the path by which the change from initial to the final state is brought about.
Some common state functions are pressure (p), volume (V), temperature (T), internal energy (U), enthalpy (H), entropy (S), etc.
Thermodynamic equilibrium
A system is said to be in thermodynamic equilibrium if its macroscopic properties (like temperature, pressure, etc.) do not change with time.
The initial state of the system corresponds to the starting state of the system in equilibrium before any type of interaction with the surroundings. In the final state, the system attains equilibrium after interaction with the surroundings. The state function gives the difference in the property of the initial state and the final state. The interaction with the surroundings means the transfer of matter or energy or both.
Types of Processes
The state of a thermodynamic system can be changed by a process. In other words, a process gives the path or operation by which a system changes from one state to another. The process may be accompanied by an exchange of matter and energy between the system and the surroundings. This results in a change in at least one of the state variables of the system.
There are certain processes in which a particular state variable (thermodynamic property of the system) is kept constant. These are identified with special names.
Some common types of processes are :
(i) Isothermal process. A process in which the temperature of the system remains constant. In such a system, heat is either supplied to the system or removed from it.
(ii) Adiabatic process. A process in which the system does not exchange heat with the surroundings, i.e., no heat leaves or enters the system. In such a process, the temperature of the system always changes.
The systems in which such processes occur are thermally insulated from the surroundings.
(iii) Isobaric process. A process in which the pressure of the system remains constant.
(iv) Isochoric process. A process in which the volume of the system remains constant.
(v) Cyclic process. A process in which the system undergoes a series of changes and ultimately returns to its original state is called a cyclic process
(vi) Reversible process. A process in which the direction may be reversed at any stage by merely a small change in a variable like temperature, pressure, etc.
Common modes of transfer of energy between system and surroundings
Transfer of energy between system and surroundings happens through the following 2 ways.
– Heat (q)
– Work (w)
Internal Energy (U)
The sum of all forms of energies stored within a substance (or a system) is called its internal energy or intrinsic energy.
– Internal energy is the sum of all types of energies, a system may have like chemical, electrical, mechanical, or any other type of energy.
– It is a state function and an extensive property
Say, UA and UB are the internal energies in states A and B respectively. Then the difference between the internal energies in the two states will be ΔU = UB – UA
The difference in internal energies (ΔU) has a fixed value and will be independent of the path taken between two states A and B.
– Absolute measurement of U is not possible so change in internal energy, ΔU is considered. ΔU = Uf – UI where Uf and UI are the internal energies of final and initial states, respectively.
– For chemical reactions, the change in internal energy may be considered as the difference between the internal energies of the products and that of the reactants, i.e., ΔU = Uproducts – Ureactants = Up – Ur where Up is the internal energy of the products, Ur is the internal energy of the reactants and ΔU gives the change in internal energy.
- If the internal energy of the products is less than the internal energy of the reactants, then ΔU would be negative.
ΔU = Up – Ur = – ve (∵ Up < Ur )
- On the other hand, if the internal energy of the products is more than the internal energy of the reactants, the ΔU would be positive.
ΔU = Up – Ur = + ve (∵ Up > Ur)
Thus, the internal energy, U is a state function.
This means that ΔU depends only on the initial and final states and is independent of the path. In other words, ΔU will be the same even if the change is brought about differently.
Sign Convention of ΔU
• If energy is released, ΔU = –ve
• If energy is absorbed, ΔU = +ve
Change of Internal Energy U of the system
Change of Internal Energy U of the system may change, when:
• Heat passes into or out of the system
• Work is done on or by the system
Heat (q) & Change of Internal Energy
It is a form of energy exchanged between the system and surroundings due to the difference in temperature between the system and surroundings.
Energy is exchanged between the system and the surroundings as heat if they are at different temperatures.
Sign Convention
• If heat is absorbed (endothermic reaction), q = +ve
• If heat is released (exothermic reaction), q = -ve
Work (w) & Change of Internal Energy
It is another mode of transference of energy. Work is said to be performed if the point of application of force is displaced in the direction of the force. It is equal to the force multiplied by the displacement (distance through which the force acts). If f is the force and dl is the displacement of the point of application in the direction in which force acts, then work done is given by w = f × dl
There are two main types of work which we generally come across. These are (i) electrical work and (ii) mechanical work.
Electrical work is important in systems where the reaction takes place between ions whereas mechanical work is performed when a system changes its volume in the presence of external pressure.
Mechanical work is important especially in systems that contain gases. This is also known as pressure-volume work.
Sign Convention (for chemistry notes)
• If work is done on the system, w = +ve (compression)
• If work is done by the system, w = -ve (expansion)
[Important: Please note that in our physics class notes on thermodynamics, we have used just the opposite convention for work, following the standard practice:
– in Physics notes, we used +ive work notation when the system does the work(expansion).
– Similarly in Physics notes, when work is done on the system (compression), we used -ive work notation.]
Work done (w) in various processes
a) Work done in irreversible isothermal expansion against a constant external pressure (pex)
w = – pex ΔV = – pex (Vf – Vi)
(-ve sign has been used as it is work of expansion i.e. work done by the system)
Vf and Vi represent the final and initial volumes, respectively
b) Work done in an isothermal reversible expansion of n moles of an ideal gas
w = – 2.303 nRT log (Vf /Vi) = – 2.303 nRT log (pi/pf)
(-ve sign has been used as it is work of expansion i.e. work done by the system)
c) Work done in Isothermal & Free Expansion (Expansion in vacuum)
The expansion of a gas in a vacuum (pex = 0) is called free expansion. It may be noted that no work is done during the free expansion of an ideal gas whether the process is reversible or irreversible.
Ideal gas : pex = O, w = O, ΔU = O (as isothermal), hence, q = O
Real gas : pex = O, w = O
• Work and heat are not state functions, they are path functions
First Law of Thermodynamics
– First Law of Thermodynamics – The energy of an isolated system is constant. Mathematically ΔU = q + w, where w is the work done on the system.
– This law is also known as the law of conservation of energy i.e. energy can neither be created nor destroyed
– For an ideal gas undergoing isothermal changes (ΔT = O), ΔU = O
Heat (q) in various processes
Heat (q) during Isothermal and Free Expansion of an Ideal Gas
pex = O, w = O, ΔU = O (as isothermal), hence, q = O
Heat (q) for isothermal irreversible change
ΔU = q+ w
Here, ΔU=O
Hence, q + w =0 => q = – w
So, q = – [ – pex (Vf – Vi) ] = pex (Vf – Vi)
Heat (q) for isothermal reversible change
ΔU = q+ w
Here, ΔU=O
Hence, q + w =0 => q = – w
So, q = – [ – 2.303 nRT log (Vf /Vi) ] = 2.303 nRT log (Vf /Vi) = 2.303 nRT log (pi/pf)
Heat (q) For adiabatic change
In this case, Heat q = o
Hence, ΔU = wad
Work, Heat & Internal energy in different processes (Infographic tabular data)
Note that here,in this chemistry notes on thermodynamics: +w = positive notation of work = work done on the system
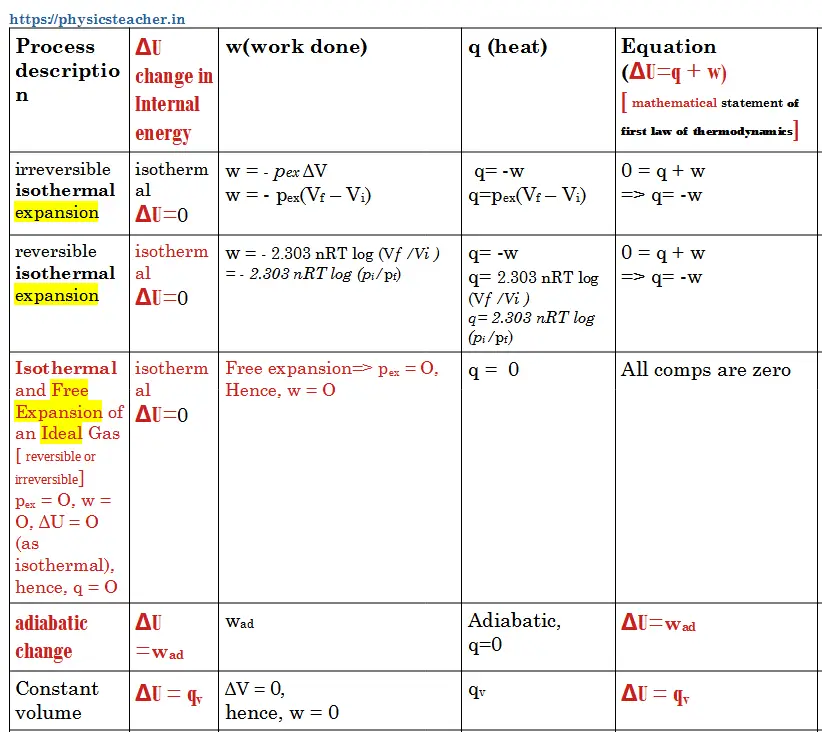